FEATURE
Investigation of Additives for MDI-based Composite and Binder Applications
Investigation of Additives for MDI-based Composite and Binder Applications
By Jason H. Stengel, Momentive Performance Materials Inc., Friendly, West Virginia
Part two of an article about the use of pMDI-based binder additive technology in wood composites, presented at the 2023 Polyurethanes Technical Conference.
This paper discloses Momentive Performance Material’s recent developments in pMDI-based binder additive technology. These developments are focused on improving processing and performance of wood composite products produced through the use of MDI-based binders. This part of the paper presents the results and discussion of the research.
Glossary and Terms
- Bin life can be defined as the time elapsed after resin has been applied to strands and prior to the strands being formed and pressed.
- Internal bond strength (IB) is a measure of the inner bond quality/strength between the strands of the panel. This property is determined via a tension force applied perpendicular to the panel surfaces. ASTM D1037, Section 11 provides details on this test.
- Water absorption testing is conducted via ASTM D1037, Section 23. This test provides methods for determining the mass and volume of water absorbed by a wood-base panel specimen of known initial moisture content (MC). Water Absorption (WA) is either recorded as mass or volume (typically both) and then reported as a percentage increase with respect to the initial MC in the specimen. Less water absorption is preferred.
- Thickness Swell (TS) is a measurement of the thickness of the panel edge or at a point one inch to the interior of the edge and is reported as a percentage increase versus the initial thickness of the panel specimen. Less swelling is preferred and indicates less water absorption.
- Moisture Content (MC) refers to the total amount of water in a piece of wood or wood-base composite and is defined as the weight of water in the wood divided by the weight of the wood. As part of this work, the MC was determined in accordance with ASTM D4442, Method A – Primary Oven-Drying Method.
- Flexural properties that were measured via procedures outlined in ASTM D1037, Section 9 include the following: Modulus of Elasticity (E) and Bending Stiffness (EI) – Panel bending stiffness is the capacity to resist deflection and is represented in bending equations as EI. The E is the apparent modulus of elasticity of the material and the I is the moment of inertia of the cross section. Traditionally in the OSB industry, the units of EI are lbf-in.2 per foot of panel width.
- Modulus of Rupture (MOR) and Maximum Moment (MM) – Bending strength capacity is the maximum moment (MM). The maximum moment of a bending specimen is calculated as the extreme fiber stress of the material (MOR) multiplied by the section modulus (S). Traditionally in the OSB industry, the units of MM are lbf-in. per foot of panel width.
- (APA Test Method) D4 Moisture Cycle is a quality control method to accelerate bond degradation commonly used in the OSB industry. The single cycle is comprised of subjecting wood-base panel specimens to a vacuum-pressure soak and redrying. The specimens are then tested dry according to the appropriate test method. In this case, D4-cycled specimens were tested in bending per ASTM D1037 in the same manner as noted for determination of dry flexural properties.
- Retained Flexural Strength is a ratio of the bending strength (MM) of the cycled (D4) and uncycled (control) specimens.
Results and Discussion
Due to the volume of information, two of the five main experiments from study 1 will be discussed in detail by analysis of the data obtained for each set of experiments following a general overview of the processing findings with and without the use of additives. The first parameter examined will be water absorption and thickness swell, followed by the internal bond strength (IB), and, finally, the findings relative to flexural properties are examined. Discussion of the results from the second study will be addressed by exploring the physical property parameters like that of study 1 with a direct comparison between each of the conditions and the performance of the panels obtained under the noted processing conditions. All data collection and specimen testing followed ASTM D1037-1211.
Experiment Overview: Processing
Each of the experimental parameters have been outlined above. Under the standard processing conditions (EXP#1), there were no noticeable processing issues with or without the use of additives. Experiment #2 provided some differentiation where a press time reduction to 140 seconds resulted in lower quality panels suffering from under cure. At 150 second press time, the panels still showed some under cure; however, with the additive UAX-1319 provided some improvement vs. no additive used. At 160 seconds press time, panels were able to be produced with and without the use of additives. Thus, a minimum press time of 160 seconds was utilized for press time reduction experiments.
The third experimental set (EXP#3) utilized a reduction in pMDI resin level from 5% to 2.5% relative to the mass of wood strands used. Here, we see no major impact on the processing, but the physical/mechanical properties were impacted particularly with the use of standard catalyst (DMDEE). The use of additive UAX-1319 and others did show improvement vs. not using the additive on certain properties such as water absorption prevention.
Experiment #4 included a combination of reduced press time and reduced pMDI loading when the 160 second press time minimum was utilized at a 2.5% pMDI loading. Here again, no processing issues were observed. However, the physical/mechanical properties were shifted due again to higher degree of under cure in the absence of additive. Standard Catalyst (DMDEE) was not compared in this study due to the issues noted with the reduced press time and typical pMDI loading.
The final experiment, #5, explored press temperature minimum at 180 second press time. The press temperature was set at 300℉ (149℃) and increased by 25℉ until under cure subsided to identify the minimum temperature for panel production. The minimum temperature was determined to be 375℉ (191℃) and only the UAX-1319 and non-additive processes were examined here. Experiment # 6 was incorporated into only a few of the noted experiments to compare the performance of the same additive UAX-1319 being added separately or as a premixed material with the pMDI and is noted as such in the tables.
With the utilization of the near standard processing conditions in study 2, all the panels produced were testable12 and there were no major processing issues. The learnings from the second study were gleaned from the physical property data and comparison of each of the 6 different conditions and their impact on the ultimate performance of the panels during testing. The following summaries for study 1 are specific to Experiment #1 and #3 and the entirety of study 2 is described (Conditions 1A – 6).
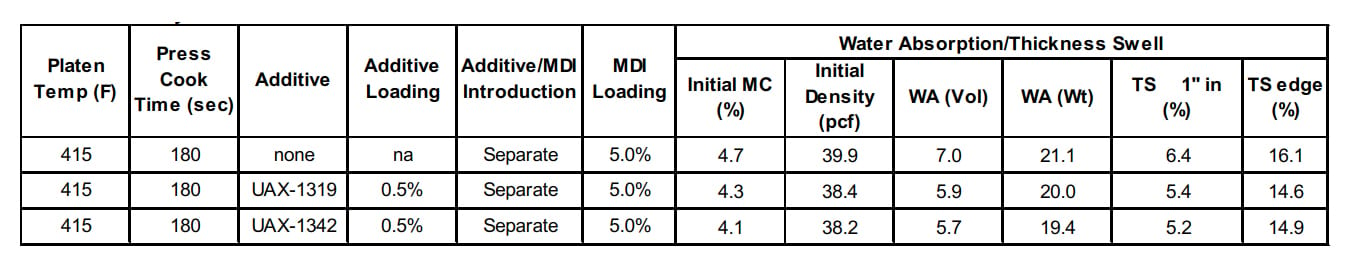
Table 4. Study 1 WA/TS Data EXP #1 Standard Process
Study 1 Results
Water absorption (WA) and thickness swell (TS) data Summary: EXP#1 and EXP #3 highlighted.
Analysis of the data shows that the use of the additive provides improvement in WA/TS properties; particularly when pushing the processing limits to reduce energy cost and improve efficiency. The pMDI level of 5% is typically utilized in high-end panels when the need for water absorption prevention is high. The lower level of 2.5% is a closer approximation to a minimum use level for OSB panel production; however, lower levels of resin are utilized in industrial production of OSB. Thus, examination of the latter parameter through the lens of water absorption may provide insights into the benefits of the additives. Density will also impact the water absorption properties as an extension of the binding properties. A properly bound panel may prevent the absorption of water more so than a poorly bound or under cured panel. In general, the density target of 38 pcf was achieved and all panels were considered comparable.
The WA/TS data for experiment #1 are noted in Table 4. Under the standard processing conditions, panels were produced without issue. The moisture properties on analysis of the test specimens show comparable initial MC; however, the TS was slightly improved over the non-additive panels and the amount of moisture taken into the specimen was also reduced with the use of additives UAX-1319 and UAX-1342.
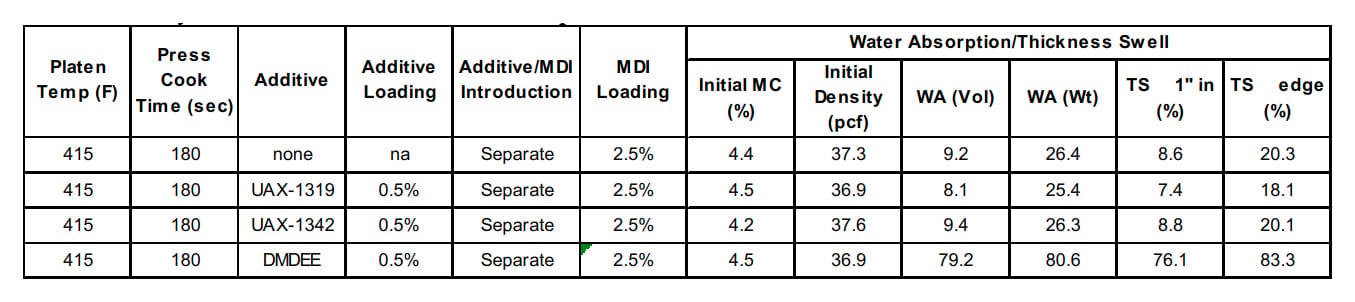
Table 5. Study 1 WA/TS data EXP #3 Reduced pMDI
The WA/TS data for experiment #3 are noted in Table 5. Reducing the pMDI load from 5% to 2.5% had a negative impact on the moisture properties when comparing to the standard conditions (EXP #1). In this case, the UAX-1319 and UAX-1342 performed similar to, or better than, the panels without additive. The use of DMDEE at this level was not beneficial for preventing water absorption.
Table 6 shows the summation of the data obtained for WA/TS analysis. The data supports a deeper look at these additives because their positive impact on water properties is noticeable even at reduced press time and temperature.
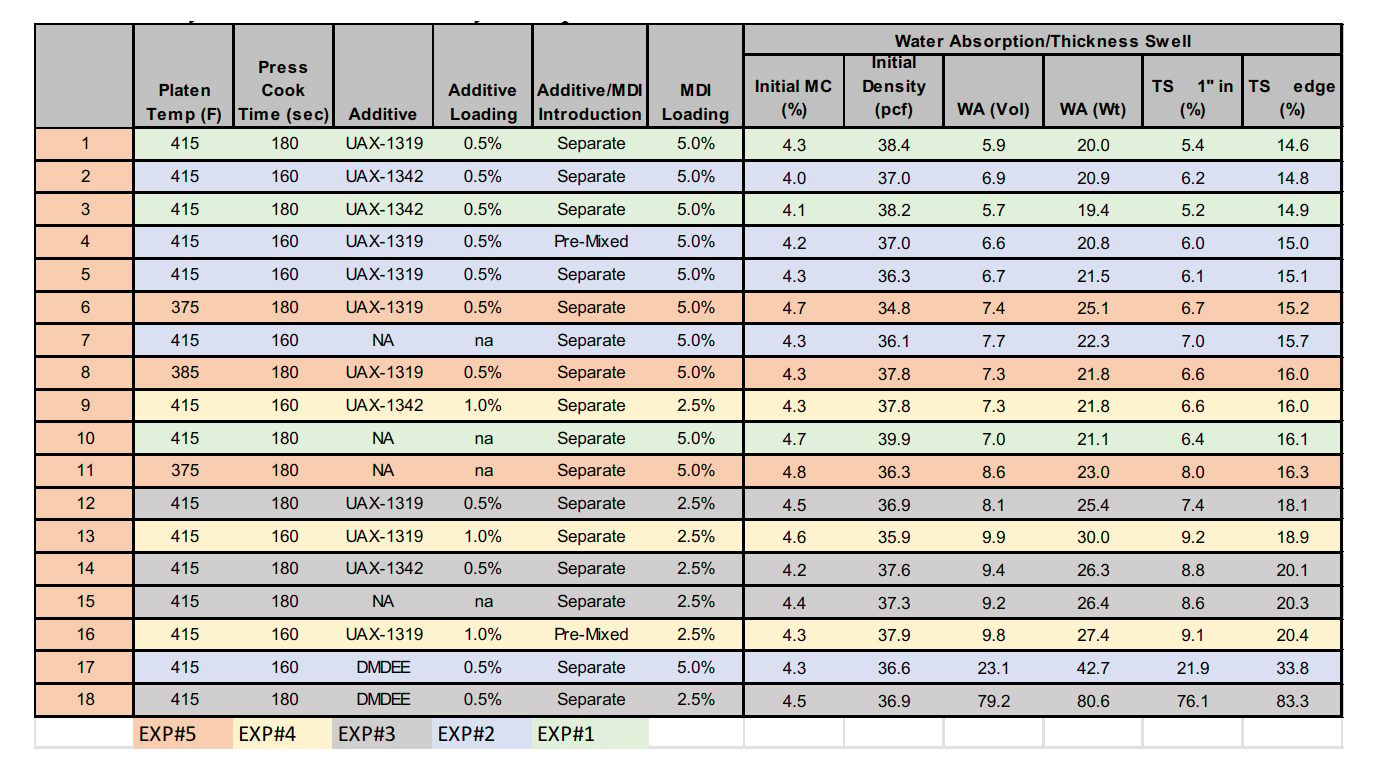
Table 6. Study 1 WA/TS Data Summary All experiments.
Table 6 also notes that the introduction of the additive, whether via combination with isocyanate ahead (row 4, 16) or via separate stream, did not make a marked difference in performance.
Internal Bond (IB) strength data Summary: EXP#1 and EXP#3 highlighted.
The IB data for experiment #1 are noted in Table 7, where we can see that UAX-1319 provides substantial improvement in IB strength vs. no additive under these processing conditions. The same panels produced with UAX-1319 and UAX-1342 also provided improvements in WA/TS. The retained flexural strength, a ratio of MM obtained from D4 cycle to that of MM dry, is substantially greater for panels produced using the additive as well.
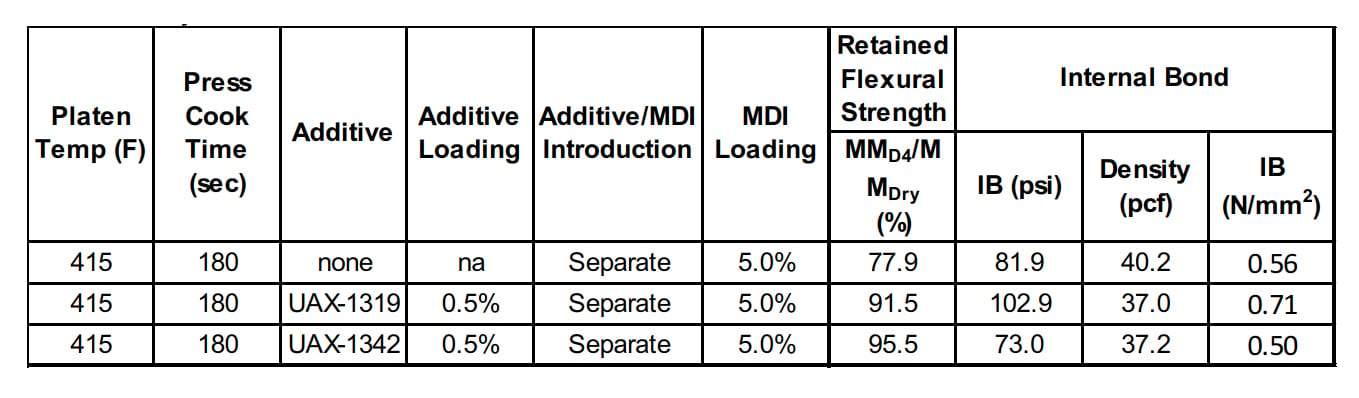
Table 7. Study 1 IB Data EXP #1 Standard Conditions.
The IB data for experiment #3 are noted in Table 8. The UAX-1319 and UAX-1342 perform well compared to the standard processed panels and are greatly improved over the performance of DMDEE at the same level. The decreased pMDI load reduced the IBs by ~44% in the case of UAX-1319 and ~25% in the case of UAX-1342, with the standard processed panels without additive being reduced by ~40%.
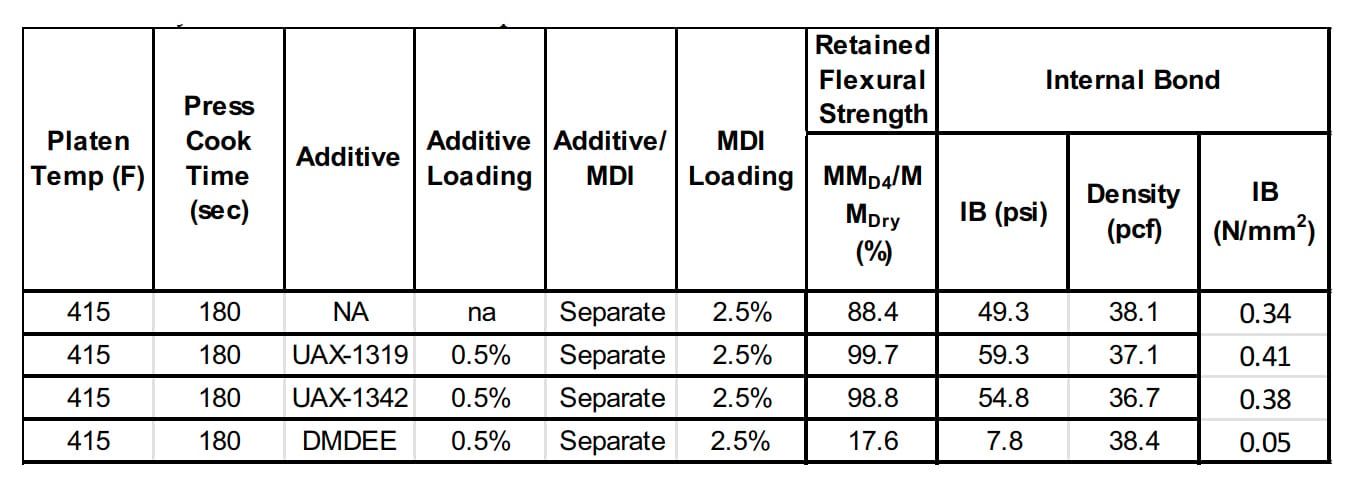
Table 8. Study 1 IB data EXP #3 Reduced pMDI.
Table 9 provides a summary of the IB data for all experiments. In general the panels produced with the experimental products outperformed the panels produced without the use of additives. DMDEE at these use levels was detrimental to the panels’ physical/mechanical properties.
UAX-1319 provides improved IB strength under all variables at a minimum of ~50 psi except for EXP#4 and EXP#5 where IB strengths of 43-48 psi were achieved. OSB grades have voluntary set minimum specifications (6). Thus, using these additives, the criteria for such grades may be met with reduction in the use level of binder resin as well as reductions in energy cost by lower temperature and time in the pressing process.
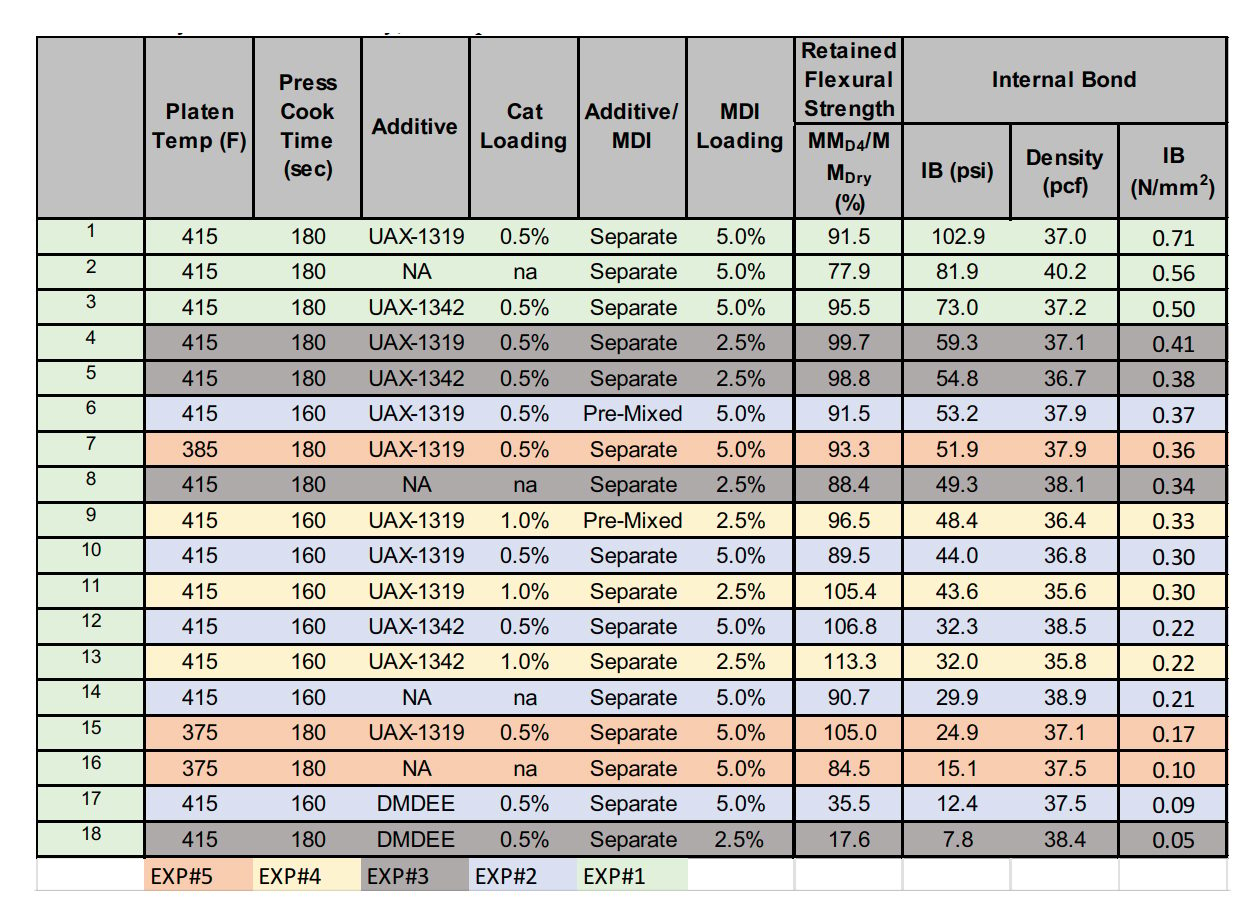
Table 9. Study 1 IB Data Summary; All Experiments.
Flexural Properties data Summary
The flexural data for experiment #1 are noted in Table 10. This analysis shows that the panels produced with the use of additives provide comparable flexural properties to that of panels produced without additive however slightly lower. The most interesting property noted is that of the retained flexural strength, a ratio of the maximum moment following D4 cycle to that of maximum moment of dry specimens, where UAX-1319 and UAX-1342 provide improvement over the non-additive panels. Typical minimum requirements for retained flexural strength in OSB are >75%. The nonadditive panels meet this criteria; the panels produced with additives UAX-1319 and UAX-1342 providing values of 91.5% and 95.5%, respectively, a substantial improvement.
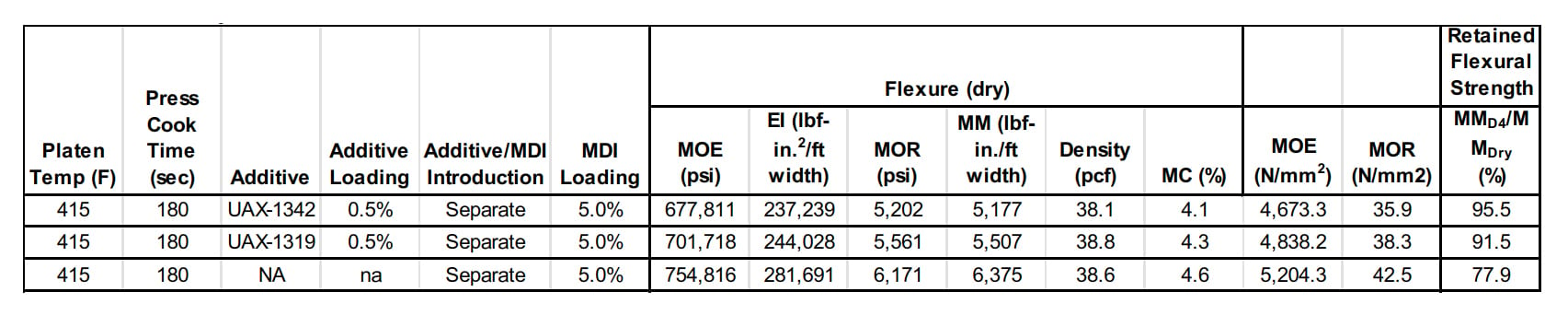
Table 10. Study 1 Flexural data EXP #1 Standard Conditions.
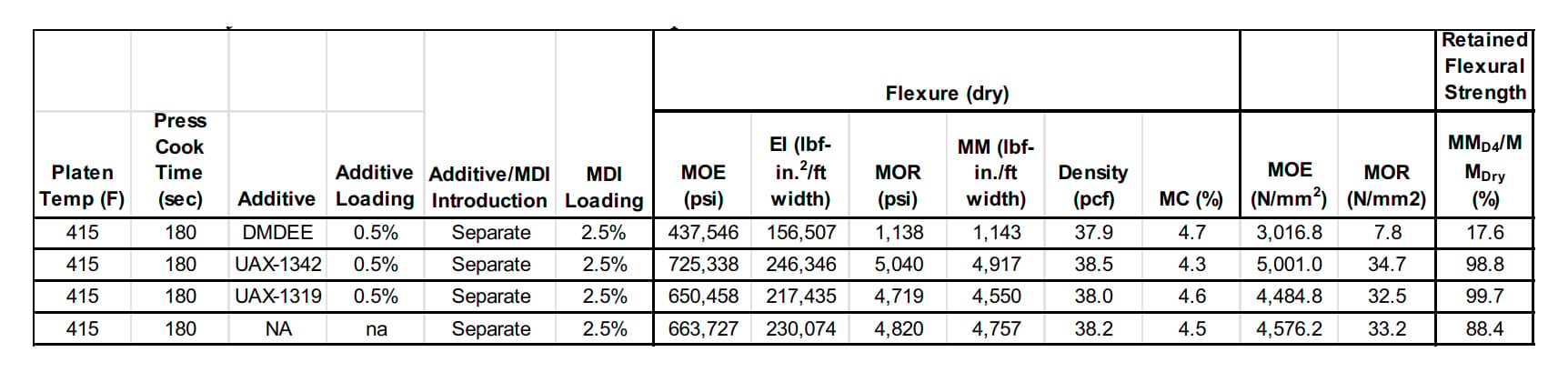
Table 11. Study 1 Flexural data EXP #3 Reduced pMDI.
The retained flexural strength noted indicates that the additives may be providing benefit and, under circumstances where the conditions for processing are more difficult i.e., lower resin loads or reduction in press time and temperature, the required physical/mechanical properties may be obtained. The summary table, Table 12, shows the flexural properties following the D4 moisture cycle.
The flexural data for experiment #3 are noted in Table 11. This is an experiment under which the conditions are more extreme. The use of 5% pMDI may be considered for use in a more demanding application where water absorption prevention and improved strength of panel are required. Here, we see that we may be able to obtain comparable physical/mechanical properties to that of the panels produced with higher resin levels with the use of additives such as UAX-1319 or UAX-1342. Again, properties are comparable for nonadditive panels to those using the experimental additives with the UAX-1342 standing out with slight improvement in MOE and MOR. Both the UAX-1319 and UAX-1342 provide excellent retained flexural strength. The panels produced with the use of DMDEE as additive again did not provide any benefit at the same use level as the experimental additives.
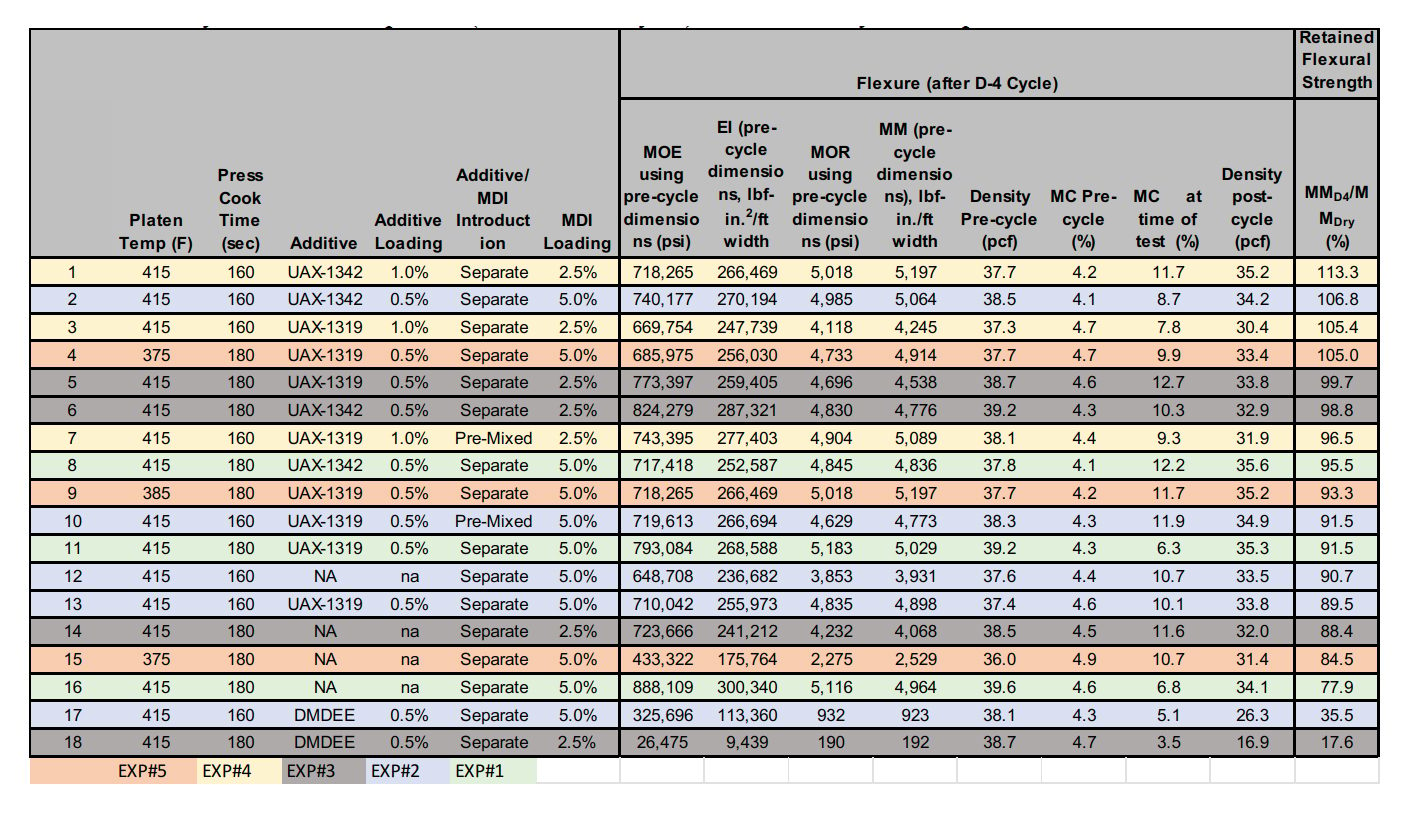
Table 12. Study 1 Flexural Properties (D4 moisture cycle) Data Summary; All Experiments.
Table 12 provides a summary of flexural properties for the panels after D4 cycle. Noting previously that the retained flexural strength was improved with the use of additives UAX-1319 and UAX-1342, this table shows the marked improvements in MOE and MOR as well as the retained flexural strength; particularly in those cases where the processing conditions were more stringent. Both UAX-1319 and UAX-1342 outperformed the nonadditive panels in nearly every case. Example 13, where UAX-1319 did not exceed the retained flexural strength of that of the nonadditive panels includes data from panels produced at 140℉ (60℃) and 150℉ (66℃) press temperatures, further exemplifying the improvements provided by the additive with a retained flexural strength of 89.5%.
Study 1 summary
We have shown that the use of additives UAX-1319 and UAX-1342 provide improvements in WA/TS properties, IB properties, and flexural properties in the production of oriented strand board. Processing conditions were modified to test the limits and capability of the additives in comparison to that of a typical additive (DMDEE) and panel production processing without additive. Under standard conditions, the additives provided benefit in moisture properties, IB, and retained flexural strength. Marked improvement is noted when the processing conditions are modified to reduce resin levels, press temperature, and press time. The results of experiment #4 in the summary table show improvements with both press cook time reduction and resin reduction when the catalyst load is increased to 1.0% b/w relative to the wood strand mass13. In the next section, we visit these topics with lower use levels of additive and compare to state-of-the-art fast-cure binder resins.
Study 2 Results and Discussion
The first study provided convincing proof of concept relative to the potential utility of the additives in processing OSB and the resulting performance advantages with respect to WA/TS, IB, and flexural properties. The standard catalysis (DMDEE) did not perform as good as the new additives and under the more difficult conditions of low temperature, reduced time at temperature, and reduced pMDI levels, the additives provided acceptable panels comparable to or improved over non-additive panels in most experiments.
The second study was initiated to identify performance advantages relative to a current state-of-the-art, "fast-cure" binder resin by comparing the properties of panels produced without additive at extended press time.
Condition 1A with extended time in press was intended to confirm that an increase of time in press would not provide the same results as additive UAX-1319. Condition 1B is a repeat of the standard conditions used in study 1. Conditions 3-5 are demonstration of the additives performance for comparison to conditions 1A, 1B, and 6. Condition 6, as noted above, was the state-of-the art comparative example.14 One major change invoked in the second study was the decrease in the use level of the additive from 10-40 wt% relative to isocyanate (0.5 wt% relative to total mass) to 0.5 wt% based on isocyanate mass, equating to ~0.025 wt% of additive based on total mass.
In the first study at the elevated use level, TS improvements up to 18% were noted compared to panels produced without the additive. Water absorption reduction improved up to 5% over no-add panels with notable improvements under the more extreme conditions such as lower temperature, shorter press time, and reduced resin load. IB data improved by 25% vs. panels produced without additive with notable improvements under the more extreme conditions. Flexural properties improved up to 22% with the use of additive compared to no-add panels.
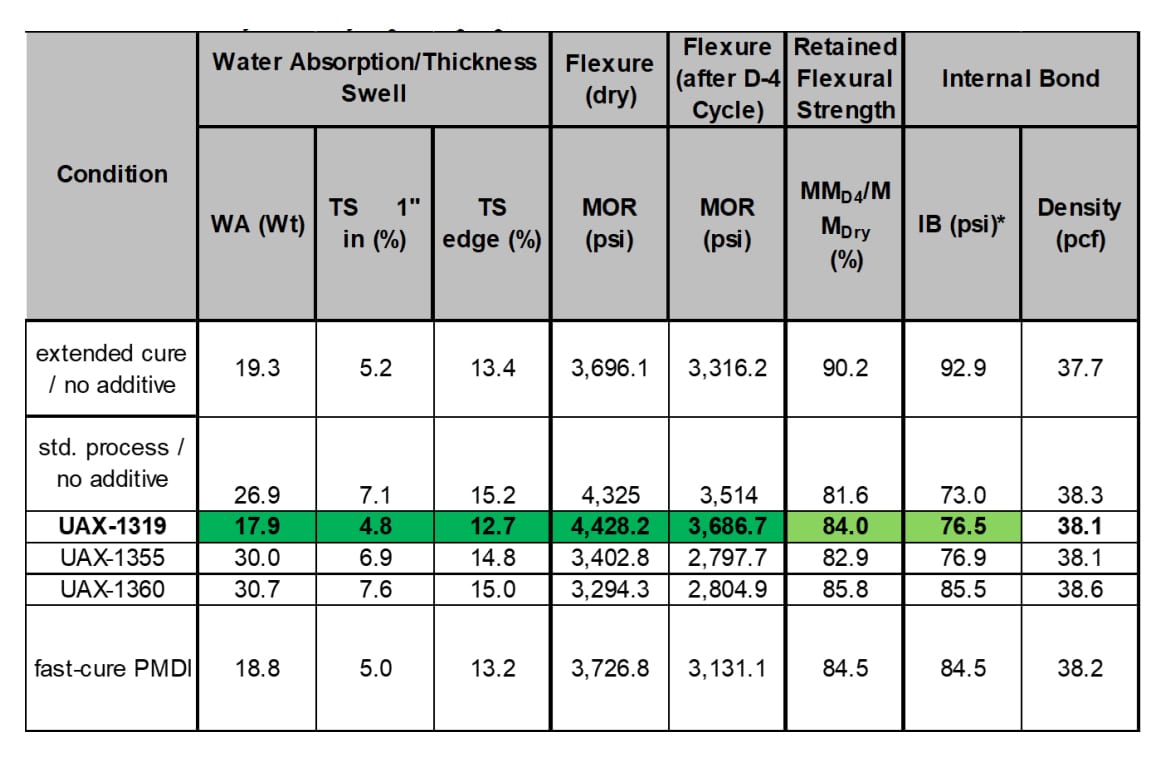
Table 13. Summary of Study 2 panel properties.
The physical property results from study 2 are detailed below. Table 13 is a summary of the data. Comparison between each of the conditions follows in subsequent tables where each of the results are compared directly to standard process panels (Condition 1B), extended press time panels (Condition 1A), and panels produced via fast-cure resin (Condition 6). We will show that the additives provide advantages in moisture and flexural properties as well as bond strength.
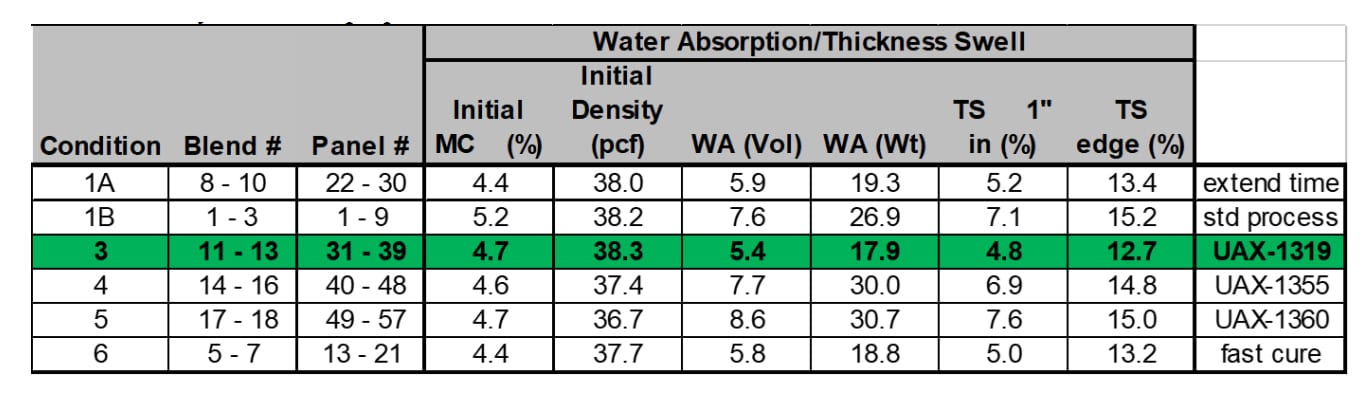
Table 14. Study 2 moisture properties.
UAX-1319 is highlighted as the standout additive; however, UAX-1360 also provides some benefit with respect to retained flexural strength and IB performance. UAX-1355 did not perform as well as either of the other two additives in this study.
Table 14 provides further detail regarding the moisture properties and highlights, again, that UAX-1319 performance, which provides substantial improvement over the standard process panels, outperforms the fast-cure resin by 3.1%.
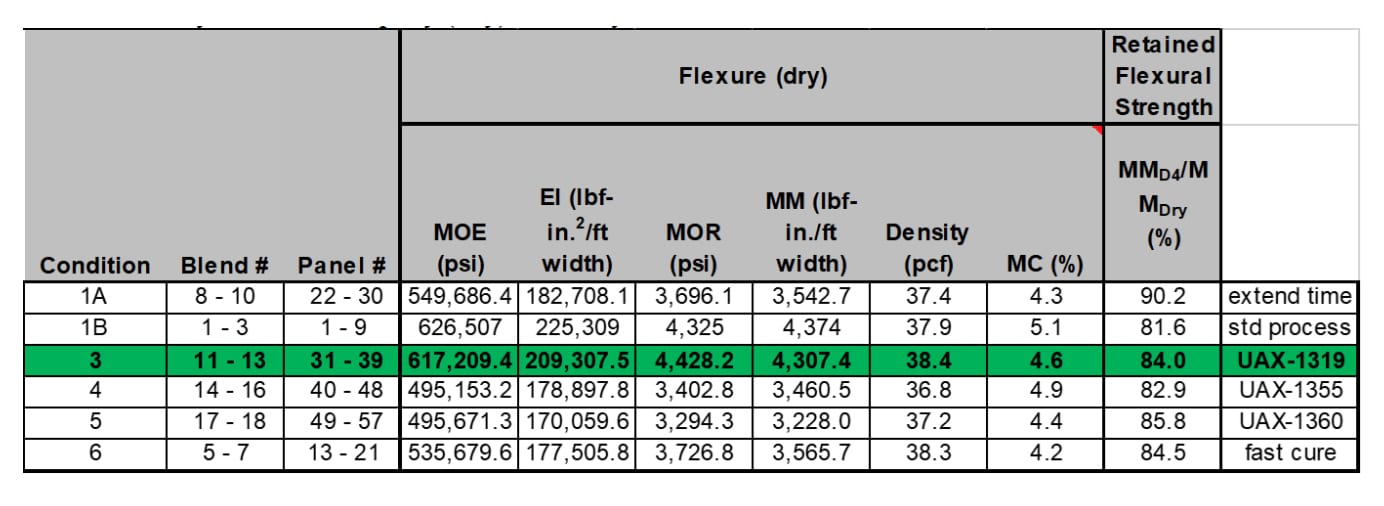
Table 15. Study 2 Flexural Property (Dry) Summary.
In this analysis, the UAX-1355 and UAX-1360 do provide improvement when compared to the standard process panels; however, they did not provide improvement when compared to extended press time and fast-cure resin.
Flexural properties were obtained with the use of dry panels as well as panels that were subjected to the D4 cycle.
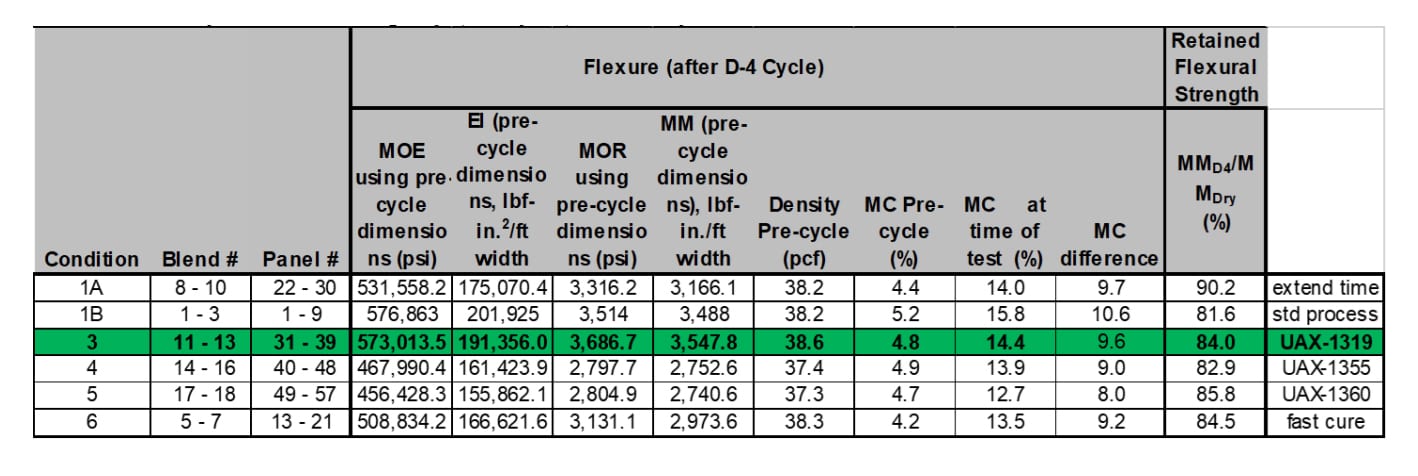
Table 16. Study 2 Flexural Property (D4 cycle) Summary.
Data obtained with dry panels is shown in Table 15. UAX-1319 provides improvements versus the extended cure and fast-cure resin panels in nearly all aspects of the flexural properties; density and moisture content are the most consistent across all conditions. The standard process panels (Condition 1B) do show a slightly higher MOE and MM, but these panels also exhibited poorer moisture properties. This performance is noticeable again in the D4 cycle (Table 16) when the panels from condition 1B have slightly higher MOE and EI values. In this case, though, the poorer moisture performance is noticeable where 1B panels increased in moisture content (MC) by a larger percentage than did the other panels.
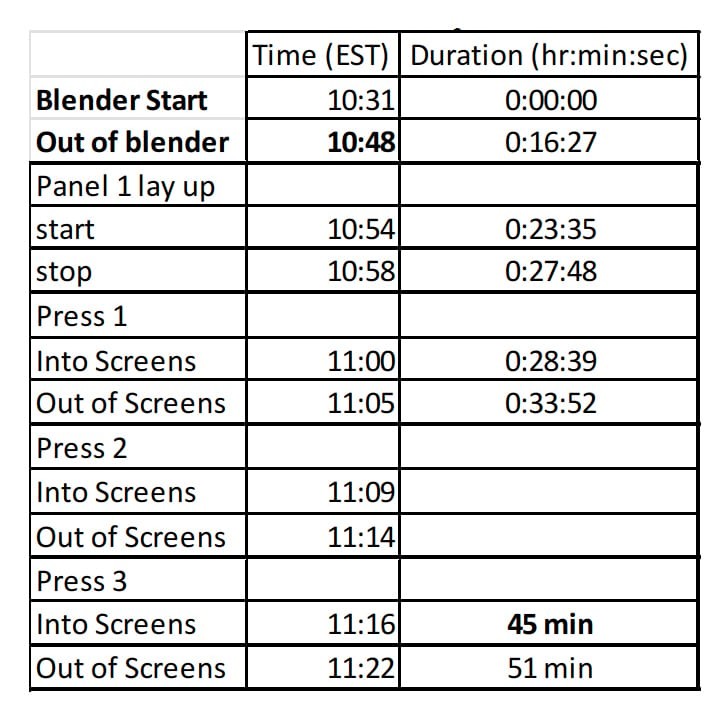
Table 17. Bin life data: Condition 3, panels 34-36.
The MOR data obtained using UAX-1319 is again a marked improvement over that of the extended press panels and the fast-cure resin panels. Retained flexural strength is comparable for all conditions.
Bin life data from study 2
As noted in the introduction and background discussion, the bin life is a critical parameter for panels produced with pMDI resin particularly those produced with ‘fast-cure’ resins as the strands typically do not go directly to the press from the blender (refer to Fig. 1). Often the material may be held in a bin following blending. As noted above, the panel production process for each condition was executed in triplicate. One blend of strands provided material to produce 3 panels. Each condition was conducted three times producing 9 panels in total, 3 panels per blend. During the production process, the strands allocated to panels 2 and 3 for the blend will remain in the bin until needed. Condition 3 identified timing for the process with the use of UAX-1319 (Table 17). We did not extend the time in the bin to further test and identify bin life. All panels processed were comparable in density and properties and some of the data for panels 34-36 are also noted (Table 18).

Table 18. Data consistency panels 34-36.
The timing for the process to produce three panels was 45 minutes from start of blending to initial pressing of the final panel. Resin addition occurred 5 minutes after starting the blender. Thus, a Bin life minimum may be estimated at 40 minutes.
The thickness and density variation of the panels indicates consistency in the process at a bin life of 40 minutes.
Conclusion
Oriented Strand Board is a frequently used material for construction. An additive composition has been identified that provides observed benefits in water absorption and thickness swell prevention along with potential benefits in processing including press time and press temperature reduction at use levels as low as 0.5% b/w resin. These benefits, demonstrated above, include 16% improvement in moisture properties (WA/TS) and improvements in Flexural properties such as MOR up to 17% when compared to properties of panels produced under similar conditions without additive as well as noticeable improvements when comparing to a fast-cure resin. The use of additives such as UAX-1319 can provide OSB production processing improvements resulting in cost savings for production facilities.
The additive that stands out in most cases is UAX-1319 because it provides improvements in most properties. The IB results were affected by reduction in use level in the second study; however, the moisture and flexural properties remained at a reduction in use level of three orders of magnitude. All the additives show stability when combined with isocyanate for extended periods of time and at elevated temperature in the absence of moisture even at levels well above 0.5% b/w resin. Furthermore, the performance of the new additives compared to DMDEE at the same use level in Study 1, and compared to a fast-cure resin in Study 2, showed benefits that can be gained in mechanical/physical property performance in addition to processing and potential cost savings.
Further studies focused on performance of these additive compositions continue. Spectroscopic analysis of panels may provide some insights into the degree of covalent bonding occurring and further studies investigating the required moisture level of the strands may also provide further cost savings benefits through reduced water consumption or through reduction in utility cost with reduction in dryer use. The full extent of the advantages that these additives may provide continues to be explored.
Part one of this paper, which presents background and experimental, can be found in the September issue of ASI.
Learn more about Momentive Performance Materials Inc. at www.momentive.com/en-us.
Acknowledgements
The authors acknowledged Brandon Doss, technician, Momentive Performance Materials Inc., and the Advanced Structure and Composites Center at the University of Maine.
"Investigation of Additives for MDI-based Composite and Binder Applications" 2023 Polyurethanes Technical Conference 25-27 September 2023, San Antonio, TX, USA. Published with permission of CPI, Center for the Polyurethanes Industry, Washington, DC.
Endnotes
11 Conducted by Advanced Structure and Composites Center at the University of Maine.
12 Testable panels were noted as not having any visual defects and the adhesion of the strands was complete without delamination.
13 Additive use level with respect to resin in this case is 40%.
14 The ‘fast-cure’ resin was obtained and used as a blind experiment.
References
1. APA - The Engineered Wood Association. [Online] 2023. [Cited: April 15, 2023.] https://www.apawood.org/technical-research.
2. Kumar, Ranjana Yadav and Jitendra. Engineered Wood Products as a Sustainable Construction Material: A Review. [ed.] Meng Gong. Engineered Wood Products for Construction. s.l. : IntechOpen, 2022, 2.
3. Natural based polyurethane matrix composites reinforced with bamboo fiber waste for use as oriented starnd board. Mariana Dias Machado Lopes, Magno de Souza Padua, Juliana Peixoto Rufino Gazem de Carvalho, Noan Tonini Simonassi, Felipe Perisse Duarte Lopez, Henry A. Colorado, Carlos Mauricio Fontes Vieira. 2021, Journal of Materials Research and Technology, pp. 2317-2324.
4. Recent progresses in wood-plastic composites: Pre-processing treatments, manufacturing techniques, recyclability and eco-friendly assessment. Ammar H. Elsheikh, Hitesh Panchal, S. Shanmugan, T. Muthuramalingam, Ahmed. M. El-Kassas, B. Ramesh. s.l. : Cleaner Engineering and Technology, 2022, Vol. 8.
5. Nishimura, T. Chipboard, oriented strand board (OSB) and structural composite lumber. [ed.] Martin P. Ansell. Wood Composites. s.l. : Elsevier Ltd, 2015, 6, pp. 103-121.
6. National Institute of Standards and Technology and U.S. Department of Commerce. Performance Standard for Wood Structural Panels. s.l. : National Institute of Standards and Technology, 2019. PS 2-18.
Opening image courtesy of BanksPhotos / iStock / Getty Images Plus.