FEATURE
Paste Adhesives for Bonding in Aerospace Applications
Paste Adhesives for Bonding in Aerospace Applications
By Dr. Ian Aspin, Adhesives R&D Scientist, Syensqo
The elements required for aircraft bonding include strength and durability, fatigue and corrosion resistance, and manufacturing flexibility.
From the ancient Egyptians using starch-based adhesives to bond papyrus to crude handmade nuts and bolts used during the Middle Ages, bonding techniques have evolved to enable humans to safely take flight into Earth’s atmosphere and beyond. But what kind of materials are required to bond massive structures that can effectively withstand extreme conditions?
Aircraft bonding is utilized for primary and secondary structures, interiors, and propulsion components. These bonding elements require high-performing attributes like withstanding extreme temperatures, strength and durability, fatigue and corrosion resistance, and manufacturing flexibility. Depending on the design, modern planes may require varied material bonding like metal-to-metal bonding, metal-to-composite bonding, composite-to-composite bonding, honeycomb sandwich bonding, cosmetic surface bonding, and others.
Aerospace manufacturers can utilize mechanical fasteners or adhesives like pastes and films. See Figure 1 for an example of the many locations bonding is required on an aircraft.
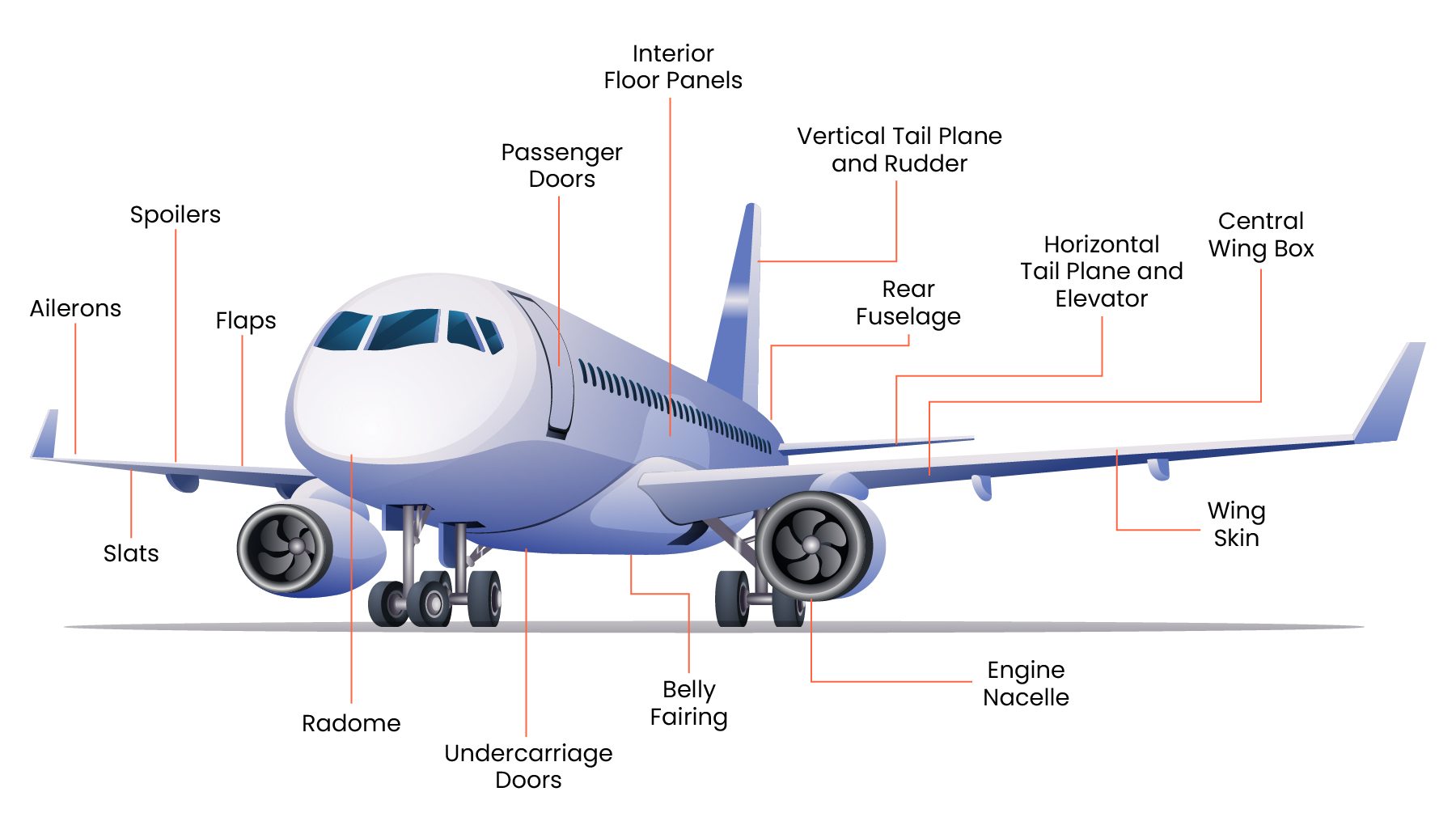
Figure 1. Many components of commercial aircraft are bonded.
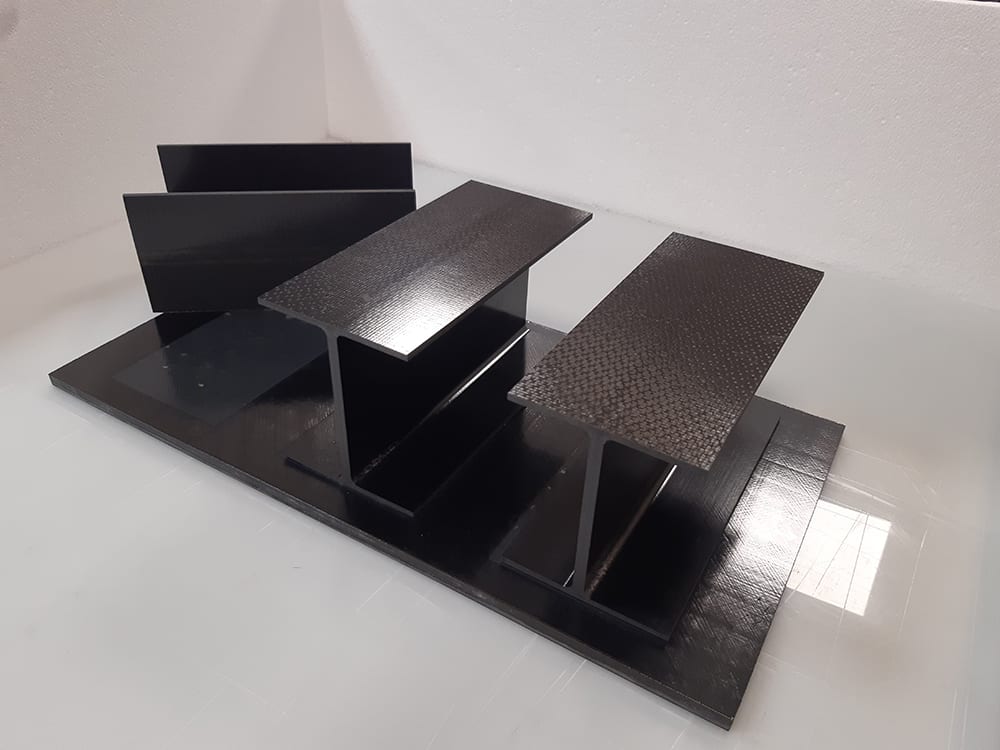
Figure 5. Resulting bonded part (I-beams to a monolithic laminate).
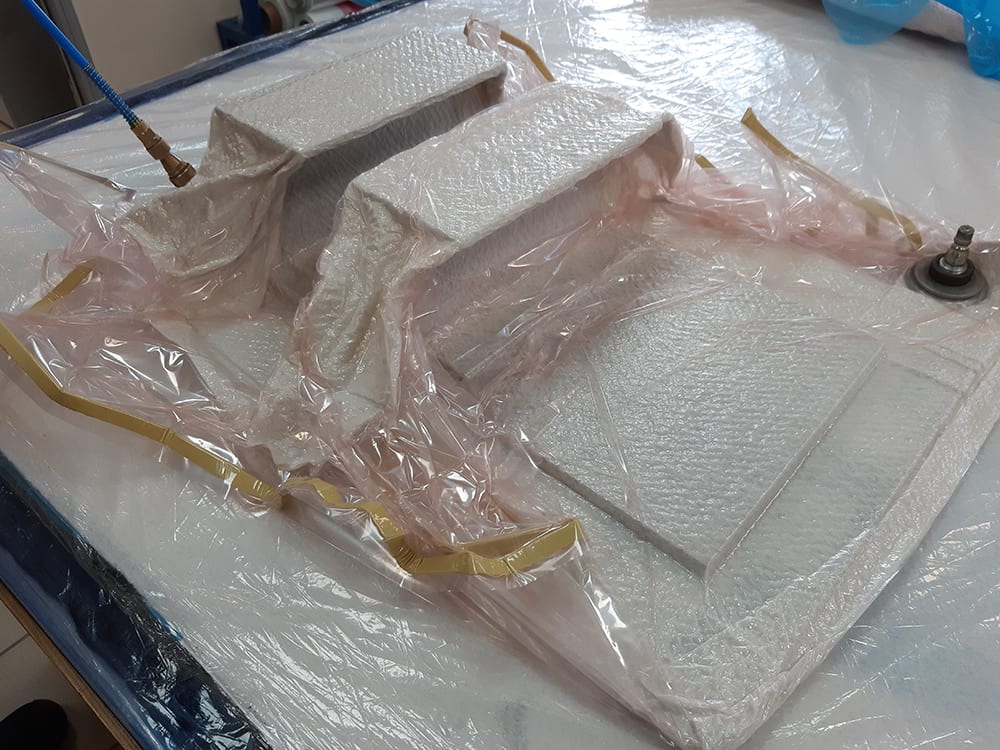
Figure 4. Vacuum-bag construction — an example of out of autoclave bonding.
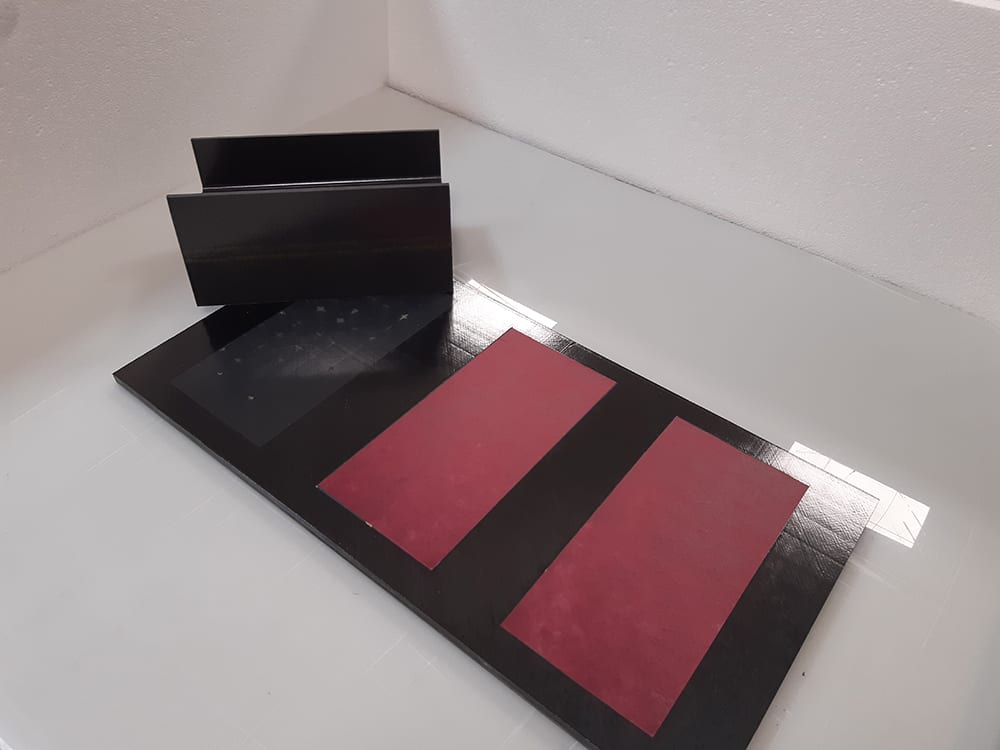
Figure 3. Assembly of adhesive and components.
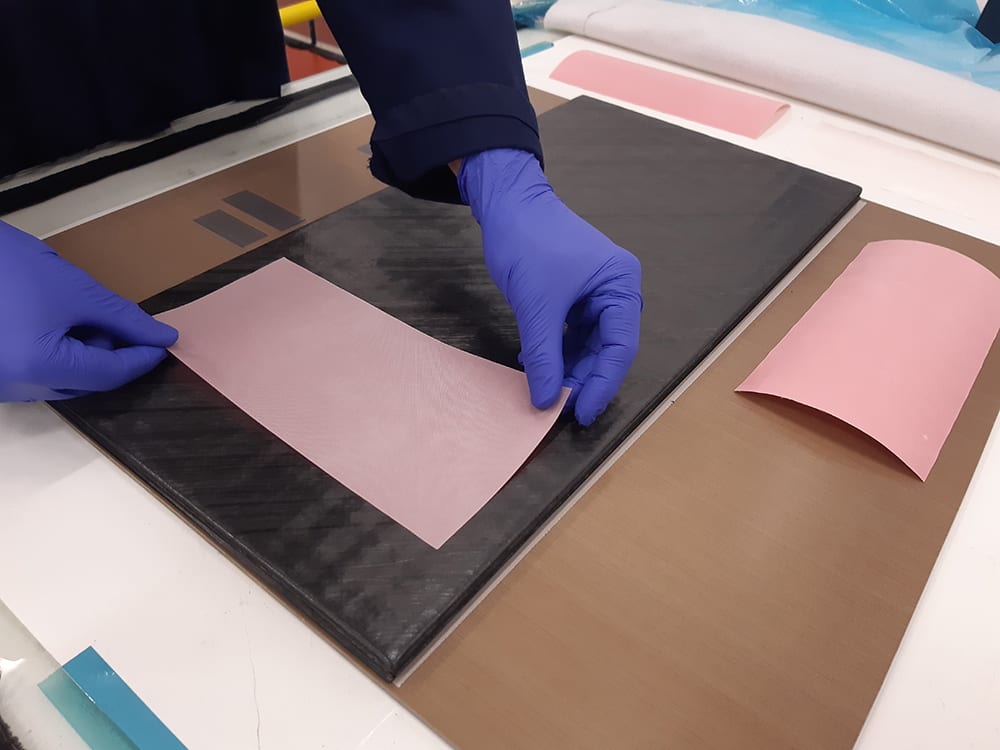
Figure 2. Adhesive film application to surface treated carbon laminate.
When considering which bonding application to use (fasteners or adhesive pastes), designers and manufacturers must consider certain tradeoffs between fasteners and adhesives.
Specific advantages of using adhesives include:
- Performance — can reduce corrosion and fatigue, allows a more uniform distribution of stress in the joint (some bonded joints are >20% stronger than riveted ones1)
- Weight savings — mechanical fasteners add weight and sometimes thickness to a part
- Faster assembly — the ease of use often means processing speeds can be increased
- Design freedom — more design options can be available when fasteners are not used
Why Bond with Pastes Instead of Films for Aerospace Applications?
Structural bonding can be achieved by using film or paste adhesives to give reliable, durable, high-performance bonds with relative ease.
Generally, film adhesives are higher performing than pastes and are obtained as uniform films of various thicknesses (areal weights) with embedded carrier scrims tailored for easy handling, bondline control, and application to large structures like commercial aircraft wings.
Manufacturers can utilize a variety of lay-up techniques designed to facilitate defect-free bonding (see Figures 2-5). However, this technique is labor intensive and time consuming.
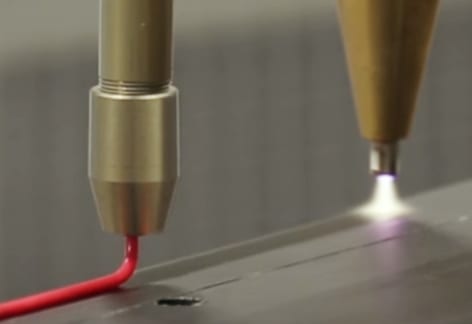
Figure 7. Automated (robotic) paste adhesive deposition.
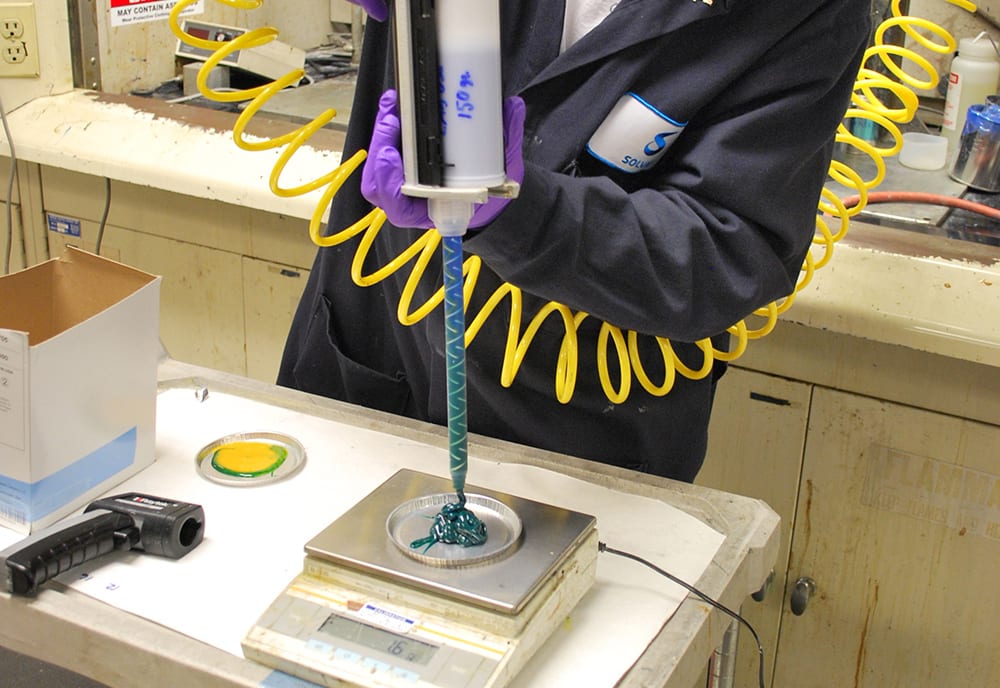
Figure 6. Pneumatic extrusion of a paste adhesive for manual application.
To enable more rapid manufacturing techniques, reduce waste and lower costs innovations in paste adhesive formulations are being driven by key attributes:
- Provide film adhesive-like performance — exhibit high strength/high toughness and excellent hot/wet environmental resistance, for bonding both metals and composite substrates
- Process flexibility — allow autoclave, out-of-autoclave, and press bonding with cure temperatures ranging from ambient conditions up to 250-350°F
- Bondline thickness tolerance — provide accurate bondlines where necessary, but also enable gap filling where required with high tensile and compression strengths
- Ease of use — exhibit thixotropy/slump resistance from a variety of application packages (by hand, cartridge, automated meter-mix pumping) where deposited adhesive displays a long pot life/assembly time to enable bonding of large structures
- Enable rapid assembly — applications with short cure times and low cure temperatures to significantly lower manufacture part cost
Pastes are generally more convenient and cost effective. While paste adhesives do require mixing and extrusion (or applied as in Figure 6), they can be automated as utilized in the automotive industry today (e.g. Figure 7). Paste adhesives can be more cost effective than film adhesives — generally, there is less scrap, using only the amount needed, and pastes can be applied by automated pumping equipment, placing material only where required. This also makes them ideal for repair or maintenance activities in the field where tooling or fixtures are absent or difficult to use.
Film adhesives, being preformulated and so stable to reacting under ambient conditions, require heat to activate, whereas paste can be formulated in two parts and activated at much lower temperatures when mixed.
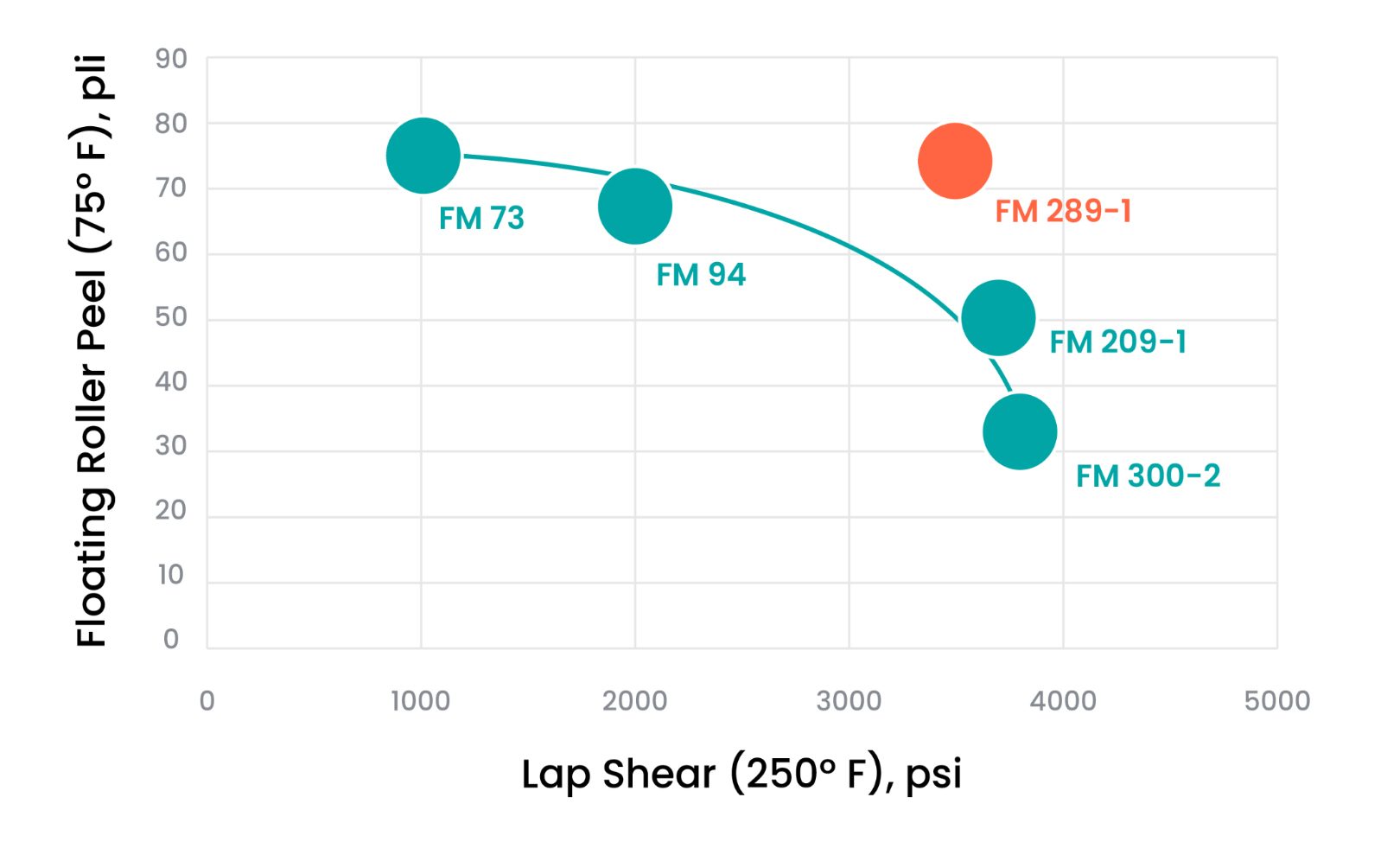
Figure 8. Peel verses lap shear properties of some 250°F cured film adhesives (data from Syensoqo).
Choosing Which Adhesive to Use — Chemistry and Properties
The choice of adhesive and the chemistry of the adhesive (epoxy, cyanate ester, acrylate, etc.) can depend upon many factors determined by the application. Cyanate ester resins have very high service temperatures (>450°F), but are expensive. Most acrylates have low temperature ceilings but are inexpensive, whilst most epoxies sit somewhere in between.
One obvious consideration is mechanical strength, but one must consider if the primary property needed is ultimate tensile strength (where little or no bending, twisting is expected) or a more flexible joint is required where resistance to the peeling apart of the substrates is necessary or some combination of both. Many of an aircraft’s control surfaces experience shear, peel, tensile, and compressive forces whilst in operation, at temperatures ranging from approximately -90 to 215°F.
The glass transition temperature (Tg), toughness, impact resistance, fatigue performance, thermal/electrical properties, environmental resistance (water, fuel, oil, solvent etc.), and finally cost are all functions of the formulation and how the individual components interact with each other.
How Strong Does the Bond Need to Be?
The demands from structural aerospace components such as wings, fuselage, and stabilizers can be very different to those of non-structural parts such as stowage bins, seats, and other interior items. A fully cured system with a high cross-link density may have a high Tg and the tensile shear strength may be considerable even at high temperatures, but this high cross-linking often induces brittleness, which in turn reduces flexibility, peel resistance, and toughness. A partially cured or low Tg system may demonstrate high flexibility due to a low cross-link density, but is often accompanied with a lower service temperature, an increased moisture absorption — attracted by unreacted functional groups — which can lead to long-term degradation of the bonded joint.
This trade-off between shear and peel performance is shown in Figure 8. Various 250°F curing film adhesives (Syensqo) showing the general relation between peel resistance (FRP) and lap shear strength (LSS).
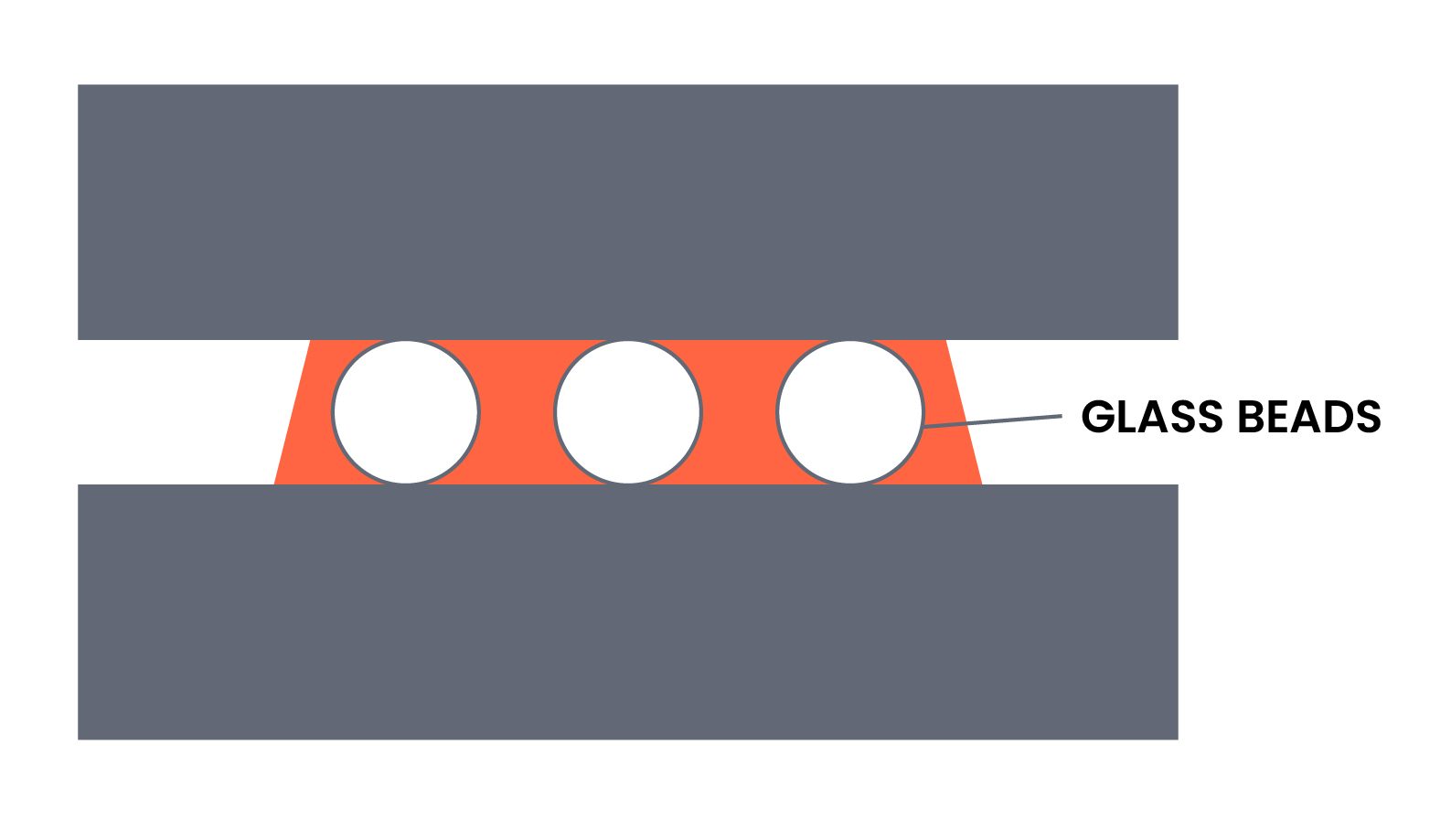
Figure 11. Schematic diagram of bondline control with glass beads.
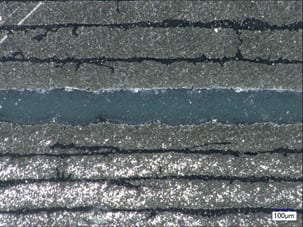
Figure 10. Cross section of a CFRP joint bonded with a paste adhesive (beads can be seen as white dots).
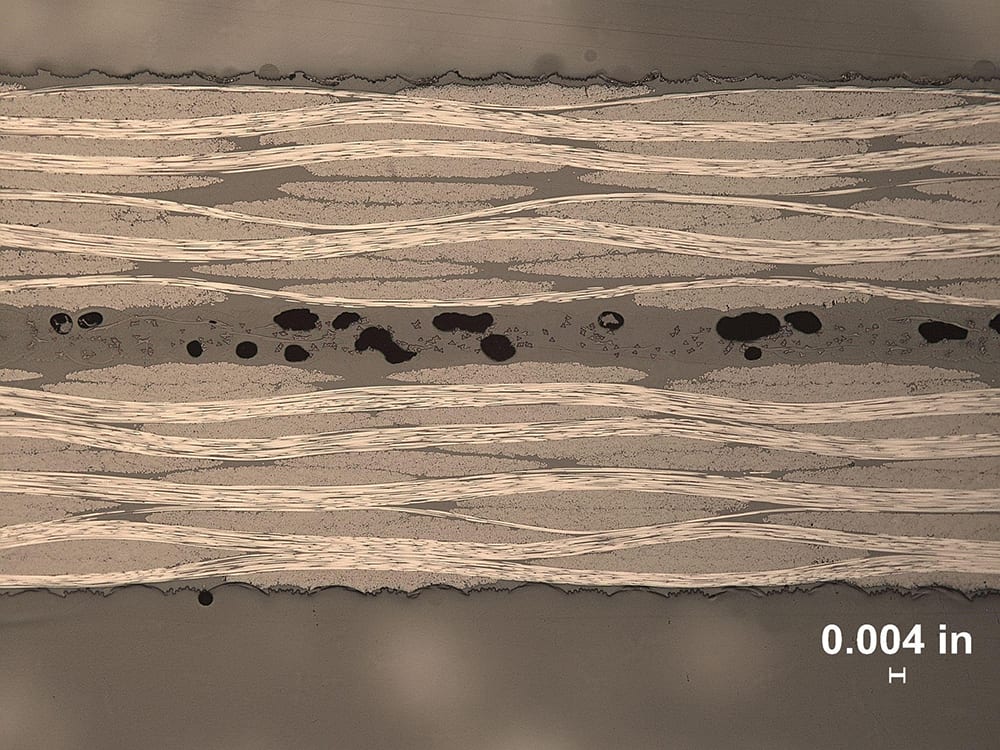
Figure 9. Cross section of a CFRP joint bonded with a film adhesive (carrier can be seen as black dots).
The adhesive formulator must choose the appropriate ingredients to give the desired properties under the constraints of the application (cure time/temperature, in-service conditions etc.). When this appears unlikely to succeed with common materials and processes, the drive to discover new materials and techniques becomes all the more important. The significant increase in the research of nanomaterials in adhesives over recent years is a good example of this, where small loadings are reported to have large beneficial effects.2
Controlling the Bondline Thickness
Once a suitable adhesive material has been identified, a number of other considerations need to be addressed, possibly none more important than how to control the bondline thickness.
Traditionally, film adhesives are supplied on a carrier (or scrim), as a woven, knitted, or random fiber (mat) fabric. Although the film can be supplied with no carrier, adhesives with carriers have an advantage in more robust handling and cutting to shape, resisting the stretching and thinning of the film to maintain thickness. The fabric can also help retain adhesive resin in the bondline gap by preventing loss by resin flow. However, when a joint is closed the carrier helps maintain a constant gap between substrates, controlling the bondline thickness (Figure 9).
When using a paste adhesive, scrims are generally not used but the gap can be maintained by the addition of a very small amount of solid glass beads of a known range of diameters. These behave as "internal shims" (Figures 10, 11) but the user has to be careful that the crush strength of the beads is suitable for the bonding pressures employed.
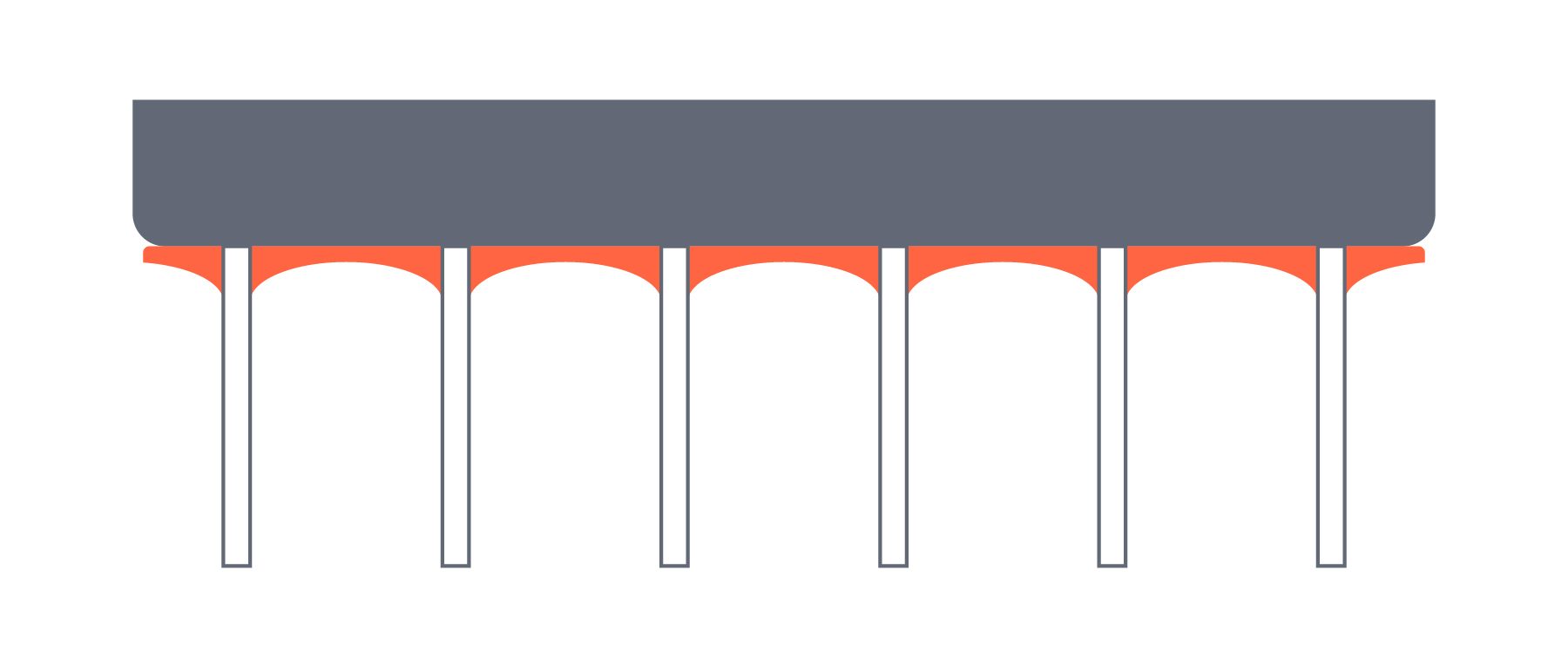
Figure 14. Schematic diagram of idealized resin fillet formation in bonded honeycomb structures.
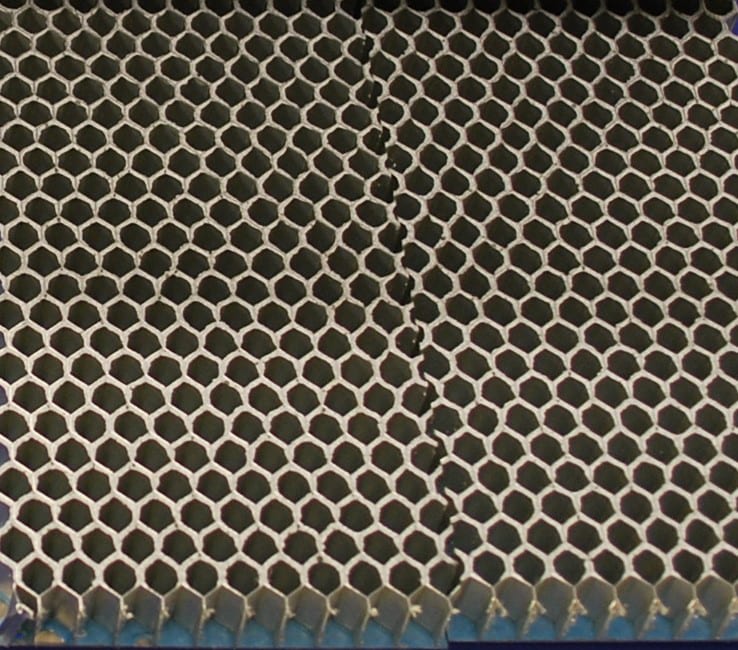
Figure 13. Examining sandwich peel coupons.
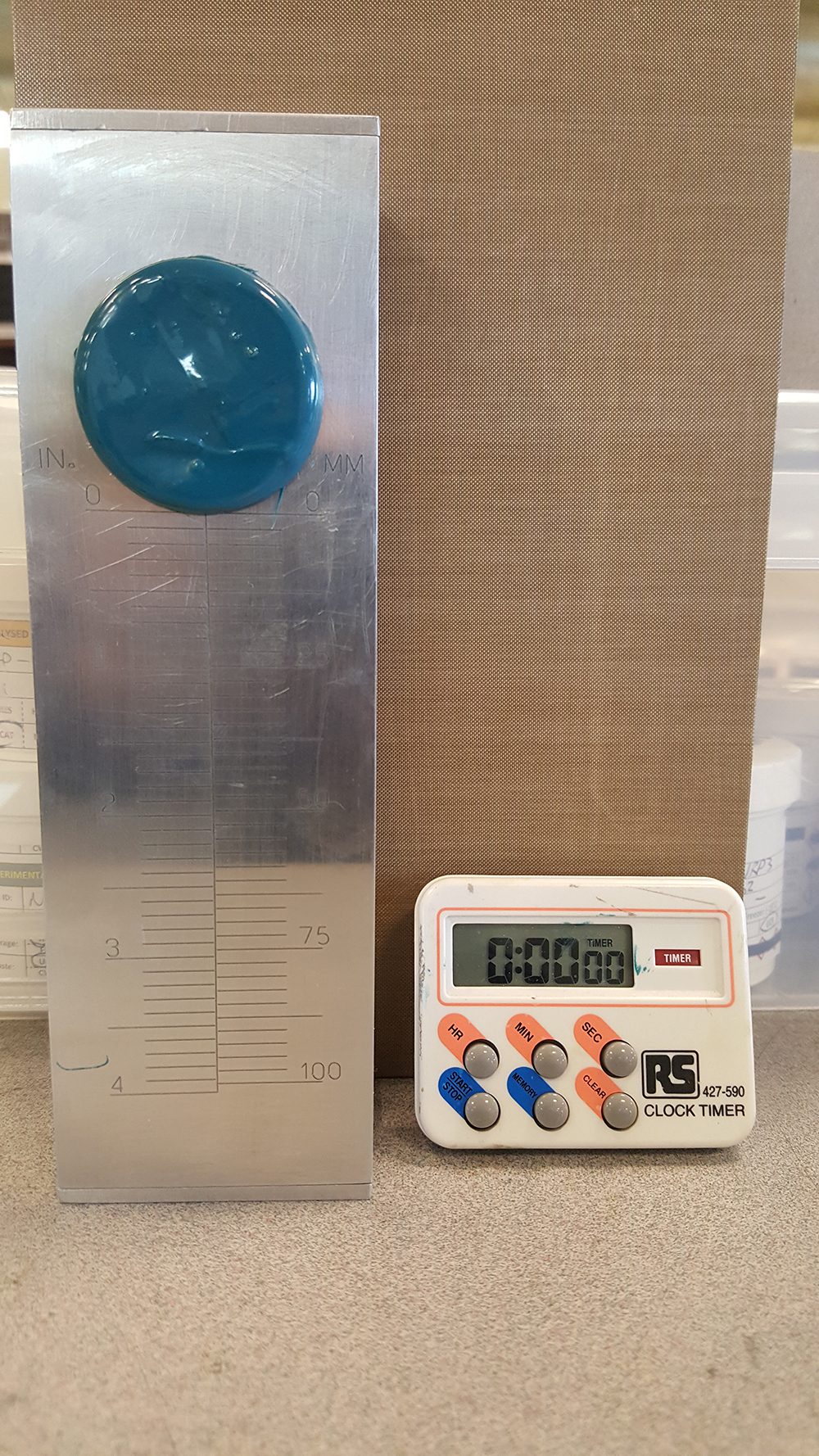
Figure 12. ASTM D2202 slump measurement.
Controlling the Flow of Paste Adhesive Resin
When closing a bonded joint and applying temperature to affect the curing chemical reactions, the resin may reduce in viscosity and there is a danger that with bonding pressure, some resin may be squeezed out, forming a resin-deficient bondline. This is reduced by the carrier and the formulating ingredients in a film adhesive, but for a paste this is entirely dependent on the formulation. This presents the formulator with a challenge: design a material that doesn’t flow (usually indicating high viscosity), yet has a low enough viscosity to allow enough flow for easy application (i.e. automated dispensing equipment), substrate surface wetting, and fill gaps on the substrate surfaces for effective bonding.
Paste Adhesive Rheology — Making Sure Your Adhesive Is Where You Want It
The phenomena of shear thinning and thixotropy are invaluable tools for the paste adhesive developer. Using the science of rheology is particularly useful to measure these characteristics and relate them to observable physical properties, such as the slump behavior (see Figure 12). A certain amount of flow is desirable — when bonding sandwich panels it is advantageous if most of the resin accumulates around the cell edges (forming good fillets) rather than remaining in the centers of the cells where it serves little purpose (see Figures 13, 14). This movement, or reticulation, of resin is dependent on the physical interaction between the adhesive and the substrate surface (surface tension and surface energy, respectively).
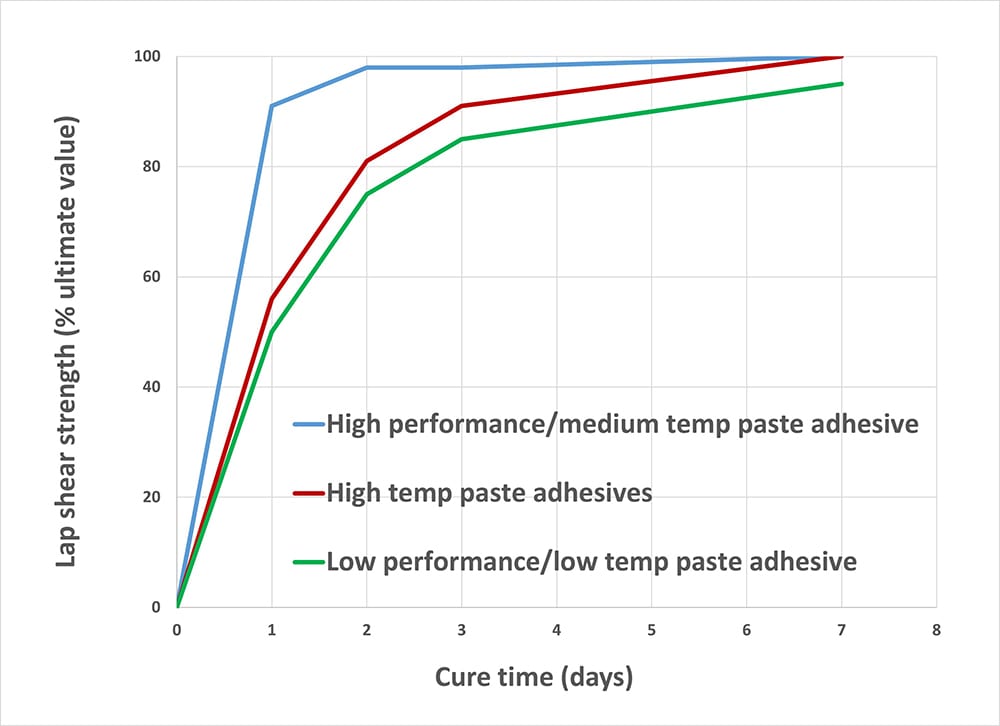
Figure 16. Rate of attaining "green strength" of three paste adhesives.
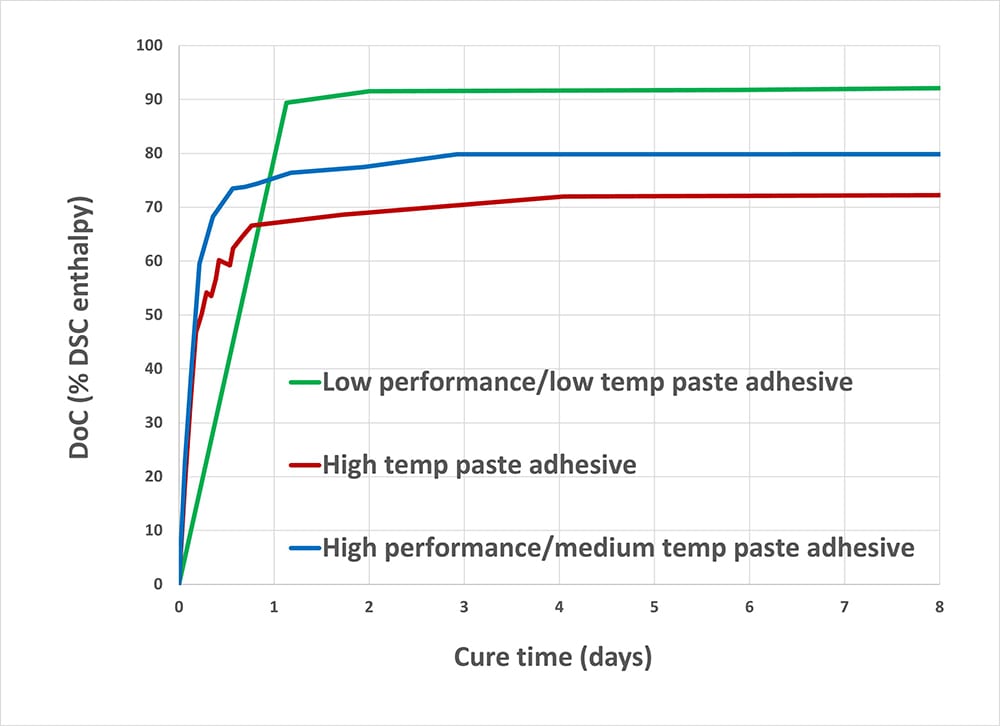
Figure 15. Rate of cure of 3 three paste adhesives (by DSC analysis).
Ambient and Post-curing Paste Adhesives — Unlocking the Potential
The use of ambient curing paste adhesives is probably the simplest method of joining well-prepared substrates, given a mixed adhesive and some fixture or jig to hold the substrates in the correct configuration whilst curing takes place. However, the user will be aware that during cure, the gel fraction increases, increasing the viscosity, surpassing the gel point and finally vitrification occurs when the Tg of the system has reached a level high enough above ambient conditions.
Further RT curing in this solidified state is very slow due to the lack of molecular mobility and diffusion. This "locked-in" structure will have a Tg that may be very low and a mechanical strength (as measured by LSS for example) below the ultimate capability compared to a fully cured system.
This initial cure mechanical strength is often called the "green" strength. For example, Figure 15 compares the rate of cure of three RT curing paste adhesives, as measured by Dynamic Scanning Calorimetry (DSC). The initial cure of two adhesives is very fast, but essentially stops at 60-75% after less than one day.
The low-performance paste on the other hand is initially slower, but reaches a higher level of conversion, due to the reactivity/functionality of the components in the formulation. Conversely, one high performance paste achieves ~90% of its ultimate LSS in one day whilst the other two systems take much longer to achieve this level as shown in Figure 16.
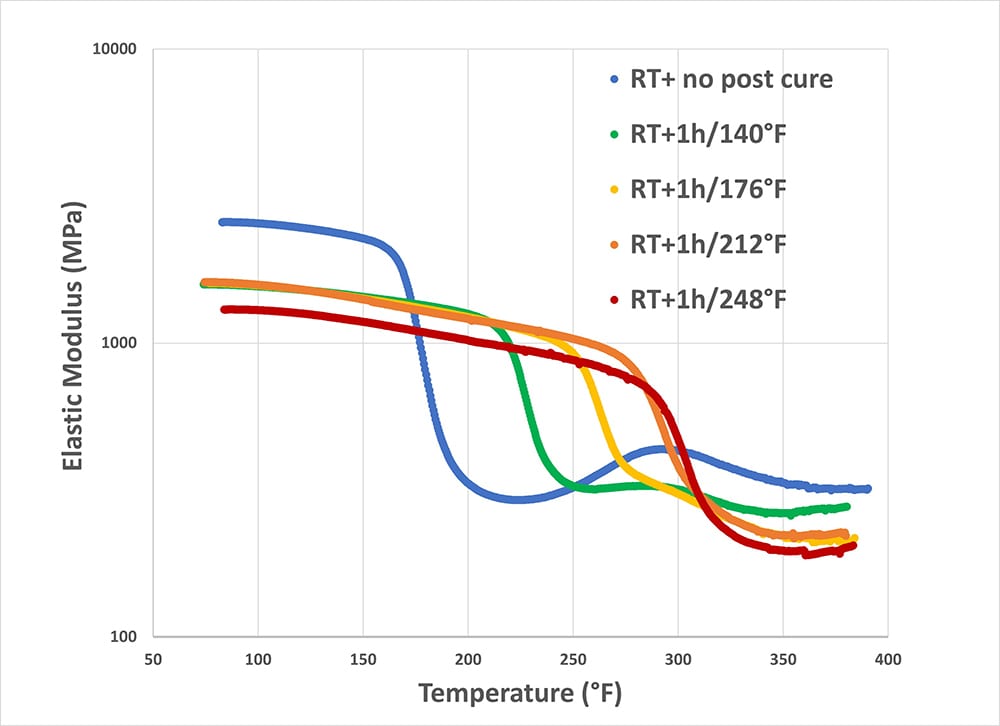
Figure 17b. Effect of post cure on the Tg of an RT cured paste adhesive.
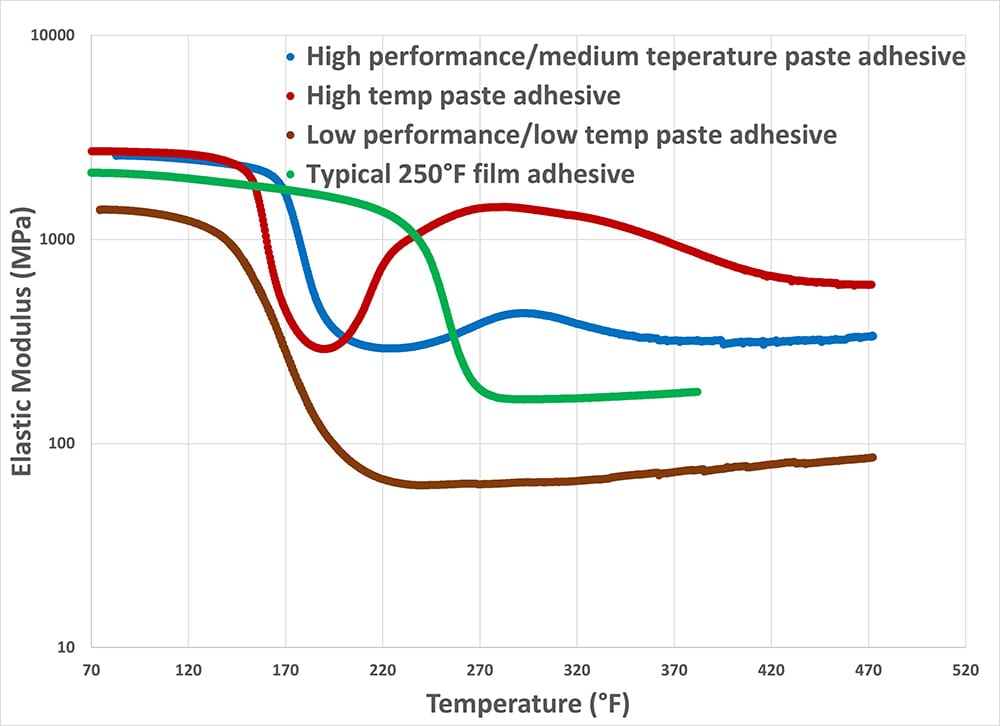
Figure 17a. Comparison of Tg behavior of some RT cured paste adhesives.
To realize the full capability of the adhesive requires a post cure, when raising the temperature above the locked-in Tg allows reactant mobility and the reaction to continue. This can be seen when evaluating ambient cured paste adhesives with Dynamic Mechanical Analysis (DMA) as in Figure 17a.
Initial ambient cure reaches a degree of conversion (DoC) of 65-75% and a Tg of around 150-170°F. Subsequent post cure treatments incrementally increase these values to near 100% conversion and a formulation dependent ultimate Tg — in the example in Figure 17b this is around 290°F.
A balance must be achieved between structure and reactivity when choosing reactive components, both resin and curing agents, bearing in mind that generally:
- Highly functional resins/curing agents react faster and generate higher Tgs and high temperature performance
- Lower functional resins/curing agents react slower, have lower Tg but are tougher
Formulating Challenges for Aerospace Applications in the Future
The challenge to the formulator of low-temperature-cure paste adhesives is to maximize strength (and Tg), toughness, and degree of cure, often using synergies between components to discover the optimum balance of properties.
Bonding with paste adhesives is not new, dating back thousands of years, but the challenges faced by formulators and manufacturers today and for the near future are for more technology-driven applications.
There is a need for greater stability of products — no refrigeration/freezer storage, longer work-life, yet contradicting this is the requirement for assemblers to generate parts in faster processes requiring faster cure times. The desire to reduce the cost of part production implies lower processing costs — lower cure temperatures, less tool-time, lower scrap rates, easier (automatable) application.
In many specialist technological applications, the incorporation of other functionalities such as electrical/thermal conductivity, enhanced damage tolerance, or indication of faults by non-destructive testing (NDT) methods means that adhesives are no longer just the "glue" holding things together.
In addition to these technical challenges are the more human and environmental aspects — the use of more sustainable or bio-sourced raw materials, ones that are less harmful to the health of suppliers, manufacturers and users, less ecologically toxic, more easily recycled resins — which all mean that there are many more developments and breakthroughs to come.
Paste adhesives have come a long way since the ancient Egyptians discovered that starch-based materials could bond papyrus and clothing, but there remain many more challenges ahead to meet the demands of bonding for future aerospace applications.
Learn more about Syensqo at www.syensqo.com.
References
1. International Journal of Adhesion and Adhesives, 2022, 113
2 Adhesives with Nanoparticles, AC Taylor in Handbook of Adhesion Technology, Eds de Silva et al, Springer, Berlin, 2011
Cover image courtesy of Jetlinerimages / iStock / Getty Images Plus.